How do plants gain mass? This intriguing question often sparks curiosity, especially among those fascinated by the wonders of the natural world. Plants, unlike animals and humans, do not consume food in the traditional sense. Instead, they rely on a remarkable process that allows them to grow and thrive, often from the tiniest of seeds to towering trees or expansive fields of crops. Understanding this process not only satisfies scientific curiosity but also underscores the essential role plants play in our global ecosystem.
The journey of a plant from a mere seed to a fully grown organism involves a complex interplay of biochemical processes, environmental factors, and genetic programming. At its core, the mass gain in plants is primarily attributed to photosynthesis, where they convert light energy into chemical energy and subsequently build the structures that make up the plant's body. This transformative process is both fascinating and vital to life on Earth, as it forms the basis of the food chain and contributes to the planet's oxygen supply.
As we delve deeper into the mechanics of plant growth, we uncover a world of interactions that include water absorption, nutrient uptake, and cellular expansion. These processes are orchestrated in harmony to enable plants to not only gain mass but also adapt to their surroundings, fend off predators, and reproduce. By exploring these mechanisms, we gain a greater appreciation for the resilience and adaptability of plants, offering insights that could enhance agricultural practices, support ecological conservation, and inspire innovations in sustainable living.
Table of Contents
- Photosynthesis: The Heart of Plant Mass Gain
- The Role of Chlorophyll in Photosynthesis
- Carbon Dioxide and Oxygen Exchange
- Water Absorption and Transport
- Nutrient Uptake from Soil
- The Process of Cellular Expansion
- The Genetic Blueprint for Growth
- Environmental Factors Influencing Plant Mass
- The Impact of Light on Plant Growth
- Temperature and Growth Rates
- Soil Quality and Its Effects
- The Role of Hormones in Plant Growth
- Adaptation and Survival Mechanisms
- The Human Impact on Plant Growth
- Future Directions in Plant Growth Research
- FAQs
- Conclusion
Photosynthesis: The Heart of Plant Mass Gain
At the core of a plant's ability to gain mass is the process of photosynthesis. This chemical process allows plants to harness energy from sunlight, transforming it into chemical energy stored in glucose molecules. Through photosynthesis, plants convert carbon dioxide from the air and water from the soil into glucose and oxygen. The glucose serves as a building block for other organic compounds, contributing to the plant's mass.
Photosynthesis occurs primarily in the leaves of plants, where chloroplasts, the specialized organelles containing chlorophyll, capture light energy. Chlorophyll’s unique structure enables it to absorb sunlight efficiently, making it the green pigment we commonly associate with healthy plants. As light is absorbed, it excites electrons in the chlorophyll, initiating a series of reactions known as the light-dependent reactions.
The light-dependent reactions produce ATP (adenosine triphosphate) and NADPH, energy-rich molecules that are then used in the Calvin cycle, or light-independent reactions, to synthesize glucose. This process not only provides the energy and organic material necessary for plant growth but also plays a crucial role in maintaining atmospheric oxygen levels, which is essential for life on Earth.
The efficiency of photosynthesis can be influenced by various factors, including light intensity, carbon dioxide concentration, temperature, and water availability. Plants have evolved to optimize photosynthesis under different environmental conditions, resulting in diverse adaptations in structure and function across species.
The Role of Chlorophyll in Photosynthesis
Chlorophyll is integral to the process of photosynthesis, acting as the primary pigment that absorbs light. Found in the chloroplasts of plant cells, chlorophyll absorbs most efficiently in the blue and red wavelengths, reflecting green, which is why plants appear green to our eyes. There are several types of chlorophyll, with chlorophyll a and chlorophyll b being the most common in plants.
Chlorophyll molecules are organized into clusters within the chloroplasts, known as photosystems. When chlorophyll absorbs light energy, it loses an electron, which is transported through a series of proteins embedded in the thylakoid membrane of the chloroplast. This electron transport chain facilitates the production of ATP and NADPH, which are essential for the synthesis of glucose in the Calvin cycle.
The presence and efficiency of chlorophyll can be affected by several factors. For instance, nitrogen deficiency in soil can lead to reduced chlorophyll production, resulting in pale or yellow leaves, a condition known as chlorosis. Additionally, environmental stressors such as high light intensity or pollution can damage chlorophyll and impair photosynthesis.
Understanding the role of chlorophyll not only helps us comprehend how plants gain mass but also informs agricultural practices. By optimizing conditions for chlorophyll production and function, farmers and gardeners can enhance crop yields and plant health. Moreover, research into artificial photosynthesis and chlorophyll analogs holds promise for renewable energy solutions, reflecting the profound impact of this pigment beyond the plant kingdom.
Carbon Dioxide and Oxygen Exchange
Carbon dioxide (CO2) and oxygen (O2) exchange in plants is a critical component of photosynthesis and respiration, two fundamental processes in plant biology. During photosynthesis, plants take in CO2 from the atmosphere through small openings in their leaves called stomata. This CO2 is then used in the Calvin cycle to produce glucose, with oxygen being released as a byproduct.
The opening and closing of stomata are regulated by the plant to balance CO2 intake and water loss. Guard cells surrounding each stoma change shape in response to environmental cues such as light, humidity, and internal CO2 concentration, ensuring that gas exchange is optimized for photosynthesis while minimizing water loss through transpiration.
In addition to photosynthesis, plants also undergo respiration, a process that occurs both day and night. During respiration, glucose is broken down in the presence of oxygen to release energy for cellular activities, with CO2 and water as byproducts. This process is essential for the plant's growth, maintenance, and reproduction, as it provides the energy required for various metabolic functions.
The interplay between photosynthesis and respiration ensures that plants can effectively gain mass and sustain their growth. By absorbing CO2 and releasing O2, plants also contribute to the global carbon cycle and play a vital role in mitigating climate change. Understanding these processes provides insights into how plants can be used in carbon sequestration strategies and highlights the importance of preserving plant biodiversity for ecosystem stability.
Water Absorption and Transport
Water is essential for plant life, serving as a solvent for nutrients, a medium for biochemical reactions, and a component of cellular structure. The absorption and transport of water in plants are facilitated by the roots and the vascular system, enabling plants to gain mass and maintain physiological functions.
Water uptake begins at the roots, where root hairs increase the surface area for absorption. The absorbed water travels through the root cortex to the xylem, a specialized tissue that transports water and dissolved minerals from the roots to the rest of the plant. This upward movement of water, known as the transpiration stream, is driven by the evaporation of water from the leaves, creating a negative pressure that pulls water upward.
The cohesion-tension theory explains how water molecules, held together by cohesive forces, move through the narrow xylem vessels. Adhesion between water molecules and the walls of the xylem vessels also aids in this upward movement. This efficient transport system ensures that water and nutrients reach all parts of the plant, supporting growth and development.
Water also plays a crucial role in photosynthesis, providing the hydrogen atoms needed to synthesize glucose and releasing oxygen as a byproduct. Additionally, water contributes to turgor pressure, which maintains cell structure and drives cellular expansion. Adequate water supply is therefore essential for optimal plant growth, and water stress can lead to reduced growth and productivity.
Understanding water transport in plants has significant implications for agriculture and horticulture. Efficient irrigation practices, soil management, and the development of drought-resistant crops are essential strategies to ensure sustainable plant growth and food security in the face of changing climate conditions.
Nutrient Uptake from Soil
Nutrients are vital for plant growth, providing the building blocks for cellular structures and metabolic processes. Macronutrients, such as nitrogen, phosphorus, and potassium, are required in large quantities, while micronutrients, including iron, magnesium, and zinc, are needed in smaller amounts but are equally important for plant health.
Plants absorb nutrients from the soil through their root systems. Root hairs, located near the root tips, enhance the surface area for nutrient absorption. Nutrients are taken up either passively, following the concentration gradient, or actively, using energy to transport ions against the gradient via carrier proteins in the root cell membranes.
The availability of nutrients in the soil is influenced by several factors, including soil pH, texture, and organic matter content. For instance, acidic soils can limit the availability of phosphorus, while alkaline soils may restrict the uptake of iron and manganese. Soil microorganisms, such as mycorrhizal fungi and nitrogen-fixing bacteria, also play a crucial role in nutrient cycling and availability.
To ensure optimal nutrient uptake, plants have evolved various mechanisms, such as root exudates that alter soil pH or chelate nutrients, making them more accessible. Additionally, plants can form symbiotic relationships with soil microbes to enhance nutrient acquisition, particularly in nutrient-poor environments.
Understanding nutrient uptake and soil-plant interactions is essential for managing crop nutrition and improving agricultural productivity. Soil testing, fertilization strategies, and sustainable farming practices are crucial for maintaining soil health and ensuring that plants receive the nutrients they need to grow and gain mass.
The Process of Cellular Expansion
Cellular expansion is a fundamental aspect of plant growth, contributing to the overall increase in plant mass. This process involves the enlargement of individual cells, driven primarily by water uptake into the central vacuole, a large fluid-filled organelle within plant cells.
As water enters the vacuole, it generates turgor pressure, which pushes the cell membrane against the rigid cell wall. This pressure causes the cell to expand, a process that is tightly regulated by the plant's growth hormones and genetic programming. The cell wall must be flexible enough to allow expansion while maintaining structural integrity, a property achieved through the dynamic remodeling of cellulose and other wall components.
Several hormones play key roles in regulating cellular expansion, with auxin being one of the most important. Auxin stimulates cell elongation by loosening the cell wall and facilitating water uptake. Other hormones, such as gibberellins and cytokinins, also contribute to cell division and elongation, working in concert to coordinate growth throughout the plant.
The rate and extent of cellular expansion can be influenced by environmental factors, including light, temperature, and water availability. Plants have evolved various adaptations to optimize growth under different conditions, such as altering leaf orientation to maximize light capture or adjusting root growth in response to soil moisture.
Understanding the mechanisms of cellular expansion provides insights into how plants gain mass and adapt to their environment. This knowledge is valuable for agriculture, where manipulating growth conditions and hormone levels can enhance crop yields and quality. Additionally, research into plant cell wall structure and expansion could lead to advances in bioengineering and the development of renewable materials.
The Genetic Blueprint for Growth
The genetic blueprint for plant growth is encoded in the plant's DNA, which contains the instructions for building and maintaining the complex structures and functions necessary for life. This genetic information is organized into genes, each of which codes for specific proteins or regulatory molecules that govern various aspects of growth and development.
Plant growth is a highly coordinated process that involves the activation and repression of specific genes at different stages of the plant's life cycle. This genetic regulation ensures that growth occurs in response to environmental cues and internal signals, allowing the plant to adapt to changing conditions and optimize resource use.
Key growth processes regulated by genes include cell division, differentiation, and elongation, as well as the synthesis of hormones, enzymes, and structural proteins. Genes also control the formation of specialized tissues and organs, such as leaves, stems, roots, and flowers, enabling the plant to perform essential functions like photosynthesis, nutrient uptake, and reproduction.
Advances in molecular biology and genomics have provided valuable insights into the genetic basis of plant growth. Techniques such as gene sequencing, functional genomics, and genetic engineering have allowed scientists to identify and manipulate specific genes involved in growth and development. This knowledge has led to the development of genetically modified crops with improved traits, such as increased yield, disease resistance, and stress tolerance.
Understanding the genetic blueprint for growth is crucial for advancing agricultural practices and addressing global challenges such as food security and climate change. By harnessing the power of genetics, researchers and breeders can develop new crop varieties that are more resilient, productive, and sustainable, ensuring a stable food supply for future generations.
Environmental Factors Influencing Plant Mass
Environmental factors play a significant role in determining the growth and mass gain of plants. These factors include light, temperature, water availability, soil quality, and nutrient levels, all of which interact to influence plant physiology and development.
Light is a critical factor for photosynthesis, driving the production of glucose and the gain of plant mass. The quantity, quality, and duration of light exposure can affect photosynthetic efficiency and growth rates. For example, plants adapted to low-light environments may have broader leaves to capture more light, while those in high-light conditions may develop thicker leaves to reduce water loss.
Temperature also influences plant growth, affecting the rate of metabolic processes and enzyme activity. Different plant species have specific temperature ranges within which they grow optimally. Extreme temperatures, whether high or low, can stress plants, leading to reduced growth and productivity.
Water availability is crucial for maintaining cellular turgor pressure, facilitating nutrient transport, and supporting photosynthesis. Drought conditions can lead to water stress, causing stomata to close and reducing photosynthetic activity. Conversely, excessive water can lead to oxygen deficiency in the root zone, impairing root function and nutrient uptake.
Soil quality affects the availability of nutrients and water to plants. Soil texture, structure, pH, and organic matter content all influence root growth and nutrient absorption. Healthy soils with balanced nutrient levels and good drainage support optimal plant growth and mass gain.
Understanding how environmental factors influence plant mass is essential for managing agricultural systems and ensuring plant health. By optimizing growing conditions, farmers and gardeners can enhance crop yields and quality. Additionally, research into plant-environment interactions can inform strategies for mitigating the impacts of climate change and supporting sustainable agriculture.
The Impact of Light on Plant Growth
Light is a fundamental driver of plant growth, providing the energy required for photosynthesis and influencing various physiological and developmental processes. The quality, intensity, and duration of light exposure can significantly impact plant growth and mass gain.
Plants utilize light energy to convert carbon dioxide and water into glucose and oxygen through photosynthesis. The efficiency of this process depends on the wavelength of light absorbed by chlorophyll and other pigments. Blue and red light are most effective for photosynthesis, while green light is mostly reflected, giving plants their characteristic green color.
Light intensity affects the rate of photosynthesis and, consequently, plant growth. In low-light conditions, plants may elongate their stems and produce larger leaves to capture more light. In contrast, high-light environments can lead to increased photosynthetic rates but may also cause photoinhibition if the light intensity exceeds the plant's capacity to process it.
The duration of light exposure, or photoperiod, influences plant growth and development. Many plants have evolved to respond to changes in day length, using cues from the photoperiod to regulate processes such as flowering, dormancy, and seed germination. Short-day plants, for example, flower when the day length decreases, while long-day plants flower when the day length increases.
Understanding the impact of light on plant growth is crucial for optimizing agricultural practices and improving crop yields. Techniques such as controlled lighting in greenhouses, the use of reflective mulches, and the selection of crop varieties adapted to specific light conditions can enhance plant growth and productivity. Additionally, research into light-responsive genes and pathways can inform the development of crops with improved performance under variable light conditions.
Temperature and Growth Rates
Temperature is a key environmental factor influencing plant growth, affecting the rate of metabolic processes, enzyme activity, and overall plant development. Each plant species has an optimal temperature range within which it grows best, with growth rates declining at temperatures outside this range.
Temperature affects the rate of photosynthesis and respiration, two fundamental processes in plant growth. As temperature increases, the rate of photosynthesis typically rises until it reaches an optimal point, beyond which it may decline due to enzyme denaturation and increased photorespiration. Similarly, respiration rates increase with temperature, providing energy for growth and maintenance but also leading to increased carbon loss.
Extreme temperatures, whether high or low, can stress plants and impact growth. High temperatures can cause heat stress, leading to reduced photosynthetic efficiency, wilting, and tissue damage. Low temperatures can slow down metabolic processes, reduce enzyme activity, and cause chilling or freezing injury.
Plants have evolved various adaptations to cope with temperature fluctuations, such as altering leaf orientation, developing heat or cold-resistant structures, and producing protective compounds. These adaptations enable plants to maintain growth and mass gain under variable temperature conditions.
Understanding the impact of temperature on plant growth is essential for managing agricultural systems and ensuring crop productivity. Strategies such as selecting temperature-tolerant crop varieties, optimizing planting dates, and implementing temperature control measures in protected environments can enhance plant growth and resilience to temperature extremes.
Soil Quality and Its Effects
Soil quality is a critical factor influencing plant growth and mass gain, as it affects the availability of nutrients, water, and air to plant roots. Healthy soils provide the foundation for robust plant growth, supporting optimal nutrient uptake and physiological function.
Soil texture, structure, and composition all influence plant growth. Sandy soils, for example, drain quickly and may not retain nutrients well, while clayey soils can retain water but may impede root growth due to poor aeration. Loamy soils, which have a balanced mixture of sand, silt, and clay, are often considered ideal for plant growth due to their good drainage and nutrient-holding capacity.
Soil pH affects nutrient availability and microbial activity. Acidic soils can limit the availability of essential nutrients such as phosphorus and calcium, while alkaline soils may restrict the uptake of iron and manganese. Maintaining a balanced soil pH is crucial for ensuring optimal nutrient availability and plant health.
Organic matter content is another important aspect of soil quality. Organic matter improves soil structure, enhances nutrient and water retention, and supports a diverse and active microbial community. Soil microorganisms play a vital role in nutrient cycling, decomposition, and disease suppression, contributing to overall soil health and plant growth.
Understanding the effects of soil quality on plant growth is essential for managing agricultural systems and ensuring sustainable crop production. Practices such as soil testing, organic amendments, crop rotation, and conservation tillage can enhance soil quality and support healthy plant growth. Additionally, research into soil-plant interactions can inform strategies for improving soil health and resilience in the face of environmental challenges.
The Role of Hormones in Plant Growth
Plant hormones, or phytohormones, are chemical messengers that regulate various aspects of plant growth and development. These hormones influence processes such as cell division, elongation, differentiation, and response to environmental stimuli, playing a crucial role in how plants gain mass and adapt to their surroundings.
Auxins are a group of hormones that promote cell elongation and are involved in regulating plant growth direction by responding to light and gravity. Auxins also play a role in root development, fruit ripening, and the formation of vascular tissues.
Gibberellins are another group of hormones that stimulate stem elongation, seed germination, and flowering. They work in conjunction with other hormones to regulate growth and development, influencing processes such as enzyme production and nutrient mobilization.
Cytokinins promote cell division and differentiation and are involved in regulating shoot and root growth. They also play a role in delaying leaf senescence and promoting nutrient uptake and transport.
Abscisic acid (ABA) is a hormone that regulates plant responses to environmental stress, such as drought and temperature extremes. ABA promotes stomatal closure to reduce water loss and helps plants enter dormancy during unfavorable conditions.
Ethylene is a gaseous hormone involved in fruit ripening, leaf abscission, and response to stress. It also influences cell elongation and the regulation of growth under stress conditions.
Understanding the role of hormones in plant growth provides insights into how plants gain mass and adapt to their environment. This knowledge is valuable for agricultural practices, where manipulating hormone levels can enhance crop yields and quality. Additionally, research into hormone signaling pathways can inform the development of crops with improved stress tolerance and performance under variable environmental conditions.
Adaptation and Survival Mechanisms
Plants have evolved various adaptation and survival mechanisms to cope with environmental challenges and ensure growth and reproduction. These mechanisms enable plants to optimize resource use, minimize stress, and maintain growth and mass gain under diverse conditions.
One key adaptation is the ability to adjust growth patterns in response to environmental cues. For example, plants can alter leaf orientation to maximize light capture or adjust root growth to access water and nutrients in the soil. These growth responses are often mediated by hormones and genetic regulation, allowing plants to adapt to changing conditions.
Plants also possess structural adaptations that enhance survival. For instance, some plants have developed thick waxy cuticles on their leaves to reduce water loss, while others have spines or thorns to deter herbivores. Certain plants have evolved specialized structures, such as tubers or bulbs, to store energy and nutrients for future growth.
Physiological adaptations, such as the production of protective compounds, also play a role in plant survival. Plants may produce allelochemicals to inhibit the growth of competing species or synthesize secondary metabolites to deter herbivores and pathogens. These compounds can enhance plant resistance to biotic and abiotic stressors, contributing to overall plant health and productivity.
Understanding plant adaptation and survival mechanisms provides valuable insights into how plants gain mass and thrive in their environments. This knowledge is important for conservation efforts, as it informs strategies for preserving plant biodiversity and resilience. Additionally, research into plant adaptations can inspire innovations in agriculture and environmental management, supporting sustainable practices and resource use.
The Human Impact on Plant Growth
Human activities have a significant impact on plant growth, influencing factors such as land use, climate change, pollution, and biodiversity. These impacts can have both positive and negative effects on plant growth and mass gain, shaping ecosystems and agricultural systems worldwide.
Land use changes, such as deforestation and urbanization, can alter natural habitats and reduce plant biodiversity. The conversion of forests and grasslands to agricultural land or urban areas can lead to habitat loss, soil degradation, and changes in local climate conditions, affecting plant growth and productivity.
Climate change is another major factor influencing plant growth. Rising temperatures, altered precipitation patterns, and increased atmospheric CO2 levels can affect plant physiology and development. While some plants may benefit from increased CO2 levels, others may experience stress due to temperature extremes and changes in water availability.
Pollution, including air and water pollution, can have detrimental effects on plant growth. Pollutants such as sulfur dioxide, ozone, and heavy metals can damage plant tissues, impair photosynthesis, and reduce growth rates. Water pollution, such as nutrient runoff and chemical contamination, can also affect plant health and ecosystem stability.
Human activities also influence plant growth through agricultural practices, which can have both positive and negative impacts. The use of fertilizers and pesticides can enhance crop yields but may also lead to soil degradation and pollution if not managed sustainably. Practices such as monoculture and intensive farming can reduce biodiversity and resilience, making plants more vulnerable to pests and diseases.
Understanding the human impact on plant growth is crucial for developing strategies to mitigate negative effects and promote sustainable practices. Conservation efforts, sustainable land management, and climate change mitigation can help preserve plant biodiversity and support healthy ecosystems. Additionally, research into sustainable agriculture and resource use can inform practices that enhance plant growth and productivity while minimizing environmental impact.
Future Directions in Plant Growth Research
Research into plant growth and mass gain is an ongoing field of study, with new discoveries and innovations continually shaping our understanding of plant biology and ecology. Future directions in plant growth research hold promise for addressing global challenges such as food security, environmental sustainability, and climate change.
One area of focus is the development of crops with improved traits, such as increased yield, stress tolerance, and nutrient use efficiency. Advances in genetic engineering, functional genomics, and plant breeding are enabling researchers to identify and manipulate specific genes involved in growth and adaptation, leading to the development of crops that can thrive under diverse environmental conditions.
Research into plant-microbe interactions is also gaining attention, as scientists explore the role of soil microorganisms in nutrient cycling, disease suppression, and plant health. Understanding these interactions can inform strategies for enhancing soil fertility and plant resilience, supporting sustainable agriculture and ecosystem stability.
Another area of interest is the study of plant responses to climate change, including the effects of rising temperatures, altered precipitation patterns, and increased atmospheric CO2 levels. Research into plant adaptation and stress responses can inform strategies for mitigating the impacts of climate change and supporting plant biodiversity and resilience.
Technological advancements, such as remote sensing, data analytics, and precision agriculture, are also transforming plant growth research and management. These tools enable researchers and farmers to monitor plant growth and health in real-time, optimize resource use, and improve decision-making, enhancing productivity and sustainability.
Understanding the future directions in plant growth research is essential for addressing global challenges and supporting the development of sustainable solutions. By harnessing the power of science and technology, researchers and practitioners can advance our understanding of plant biology and ecology, supporting healthy ecosystems and resilient agricultural systems for future generations.
FAQs
1. How do plants gain mass if they don't eat food like animals do?
Plants gain mass primarily through photosynthesis, where they convert light energy into chemical energy stored in glucose molecules. They absorb carbon dioxide from the air and water from the soil to produce glucose, which is then used to build plant structures.
2. What role does chlorophyll play in plant mass gain?
Chlorophyll is crucial for photosynthesis as it absorbs sunlight, which is essential for converting carbon dioxide and water into glucose. Chlorophyll's ability to capture light energy facilitates the production of the organic compounds that contribute to plant mass.
3. How do environmental factors affect plant growth and mass gain?
Environmental factors such as light, temperature, water availability, and soil quality significantly influence plant growth and mass gain. These factors affect photosynthesis, nutrient uptake, and overall plant physiology, determining growth rates and productivity.
4. Can plants adapt to changing environmental conditions?
Yes, plants have evolved various adaptations to cope with environmental changes. They can adjust growth patterns, develop protective structures, and produce compounds that enhance resistance to stressors, enabling them to survive and grow under diverse conditions.
5. How does human activity impact plant growth?
Human activities such as land use changes, pollution, and climate change can significantly impact plant growth. These activities can alter habitats, affect nutrient availability, and introduce stressors that influence plant health and productivity.
6. What are future directions in plant growth research?
Future research focuses on developing crops with improved traits, understanding plant-microbe interactions, studying plant responses to climate change, and utilizing technological advancements to enhance plant growth and sustainability. These efforts aim to address global challenges and support resilient agricultural systems.
Conclusion
Understanding how plants gain mass provides valuable insights into the fundamental processes that drive plant growth and development. From photosynthesis and nutrient uptake to environmental adaptations and genetic regulation, these processes highlight the complexity and resilience of plants. As we continue to explore the science behind plant growth, we uncover opportunities to enhance agricultural practices, support ecological conservation, and address global challenges such as food security and climate change. By appreciating the intricate mechanisms that enable plants to thrive, we can develop strategies to ensure sustainable growth and a healthy planet for future generations.

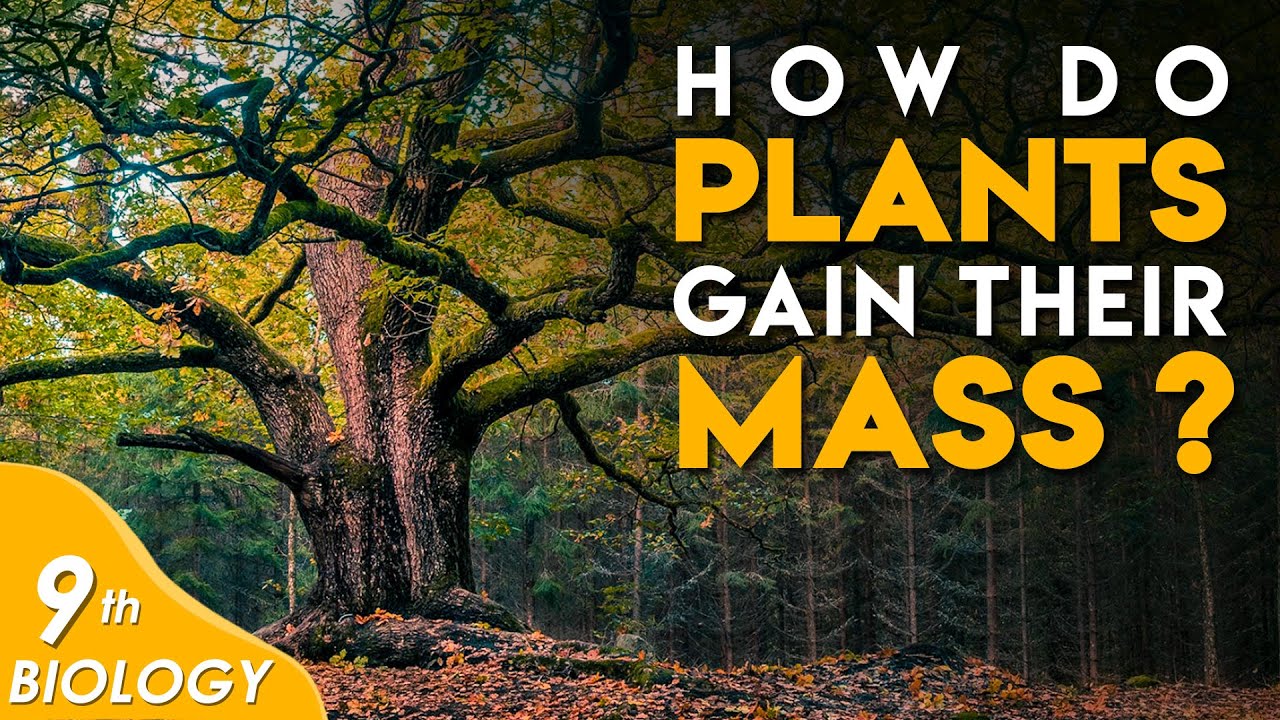