Have you ever wondered about the intricate geometrical configurations of molecules such as PH3? Understanding the electron pair geometry of a molecule like PH3 can seem daunting, yet it is a fascinating subject that unveils the hidden symmetries and chemical behaviors of molecules. This topic not only draws the curiosity of chemistry enthusiasts but also plays a crucial role in fields ranging from material science to pharmaceuticals. By delving into the nuances of PH3 electron pair geometry, we can better appreciate how these principles underpin the structures and functions of countless chemical compounds.
Phosphine, or PH3, is a simple compound that might look straightforward at first glance. However, its electron pair geometry presents a unique case study in molecular chemistry. This article aims to shed light on the subject by exploring the shape, polarity, and hybridization of PH3. We'll delve into the fundamental principles governing its structure, unravel the effects of nonbonding electron pairs, and discuss how this geometry influences its chemical properties. Whether you're a student aiming to grasp the basics or a professional seeking deeper insights, understanding PH3's electron pair geometry is crucial for a holistic view of molecular chemistry.
In this comprehensive guide, we will navigate through various aspects of PH3 electron pair geometry, unraveling its complexities while maintaining clarity and engagement. We'll cover everything from fundamental concepts to advanced applications, ensuring a thorough understanding of how PH3 behaves in different contexts. Our journey will also include frequently asked questions to reinforce key concepts and provide clarity on common queries. Get ready to explore this captivating topic, where the microscopic world of phosphine unveils its secrets and contributes to our broader understanding of chemistry.
Table of Contents
- What is PH3?
- Understanding Electron Pair Geometry
- Molecular Geometry of PH3
- Bonding in PH3
- Lone Pairs and Their Effects
- Hybridization in PH3
- Polarity of PH3
- Comparison with Other Molecules
- Applications of PH3
- Frequently Asked Questions
- Conclusion
What is PH3?
Phosphine, denoted as PH3, is a colorless, flammable, and toxic gas with a distinct, unpleasant odor reminiscent of fish or garlic. Composed of one phosphorus atom and three hydrogen atoms, it is a compound that has been recognized and utilized in various industrial and chemical processes. Phosphine is also an essential precursor in the production of semiconductors, an area where its unique properties are exploited to create advanced electronic components. Moreover, phosphine plays a role in agriculture as a pesticide, highlighting its wide-ranging applications.
From a chemical perspective, phosphine is characterized by its molecular weight of approximately 34 g/mol and its boiling point of -87.7°C. Despite its simple structure, PH3 is a molecule that provides rich insights into the study of electron pair geometry, particularly due to the presence of a lone pair of electrons on the phosphorus atom. This lone pair significantly influences the molecule's geometry and chemical behavior, making it an intriguing subject for both theoretical and practical exploration.
The study of PH3 is not limited to its applications or chemical properties; it extends to understanding its role in the broader context of molecular geometry. By examining PH3, scientists and students alike can gain a more profound comprehension of molecular interactions, hybridization, and the impact of lone pairs on chemical reactivity. As we proceed through this article, we will delve deeper into the specifics of PH3's electron pair geometry, uncovering the intricate balance between bonding and nonbonding electron pairs that define its structure.
Understanding Electron Pair Geometry
Electron pair geometry is a fundamental concept in chemistry that describes the spatial arrangement of electron pairs around a central atom in a molecule. This arrangement is crucial in determining the molecule's overall shape, reactivity, and physical properties. The geometry is influenced by both bonding electron pairs, which are shared between atoms, and lone pairs, which are not involved in bonding but occupy space around the central atom.
The Valence Shell Electron Pair Repulsion (VSEPR) theory is a widely used model for predicting electron pair geometry. According to VSEPR theory, electron pairs arrange themselves to minimize repulsion, leading to specific geometric patterns. For instance, a central atom with four electron pairs, such as in methane (CH4), adopts a tetrahedral geometry. However, the presence of lone pairs can alter this ideal geometry, as seen in molecules like PH3, where a lone pair on phosphorus changes the geometry from tetrahedral to a more pyramidal shape.
Understanding electron pair geometry is essential for predicting molecular behavior and properties. It helps chemists determine molecular polarity, reactivity, and interactions with other molecules. By applying VSEPR theory and considering the influence of lone pairs, scientists can accurately model and predict the behavior of complex molecular systems. This knowledge is not only fundamental in academic settings but also has practical implications in fields such as drug design, materials science, and environmental chemistry.
Molecular Geometry of PH3
The molecular geometry of PH3 is a fascinating example of how lone pairs influence the shape of a molecule. In PH3, the phosphorus atom is bonded to three hydrogen atoms, with a lone pair of electrons residing on phosphorus. This arrangement results in a trigonal pyramidal geometry, which is a deviation from the ideal tetrahedral shape expected if there were no lone pairs.
The presence of the lone pair on phosphorus is the key factor in determining the geometry of PH3. Lone pairs occupy more space than bonding pairs, resulting in increased repulsion. This repulsion pushes the hydrogen atoms closer together, creating a pyramidal shape rather than a flat, triangular one. As a result, the bond angles in PH3 are slightly less than the typical 109.5° found in tetrahedral molecules, usually around 93.5°.
This unique geometry has significant implications for the properties of PH3. The asymmetrical distribution of electrons and the presence of the lone pair make PH3 a polar molecule, with a net dipole moment. This polarity affects how PH3 interacts with other molecules, contributing to its reactivity and solubility in various solvents. Moreover, understanding the molecular geometry of PH3 provides insights into the behavior of similar compounds, where lone pairs play a crucial role in defining molecular structure.
Bonding in PH3
The bonding in PH3 is primarily covalent, involving the sharing of electrons between the phosphorus atom and the three hydrogen atoms. Each P-H bond is formed through the overlap of the phosphorus 3p orbitals with the 1s orbitals of hydrogen. This type of bonding is characteristic of many non-metal compounds and is essential for maintaining the structural integrity of the molecule.
In PH3, the phosphorus atom has five valence electrons, of which three are used in bonding with hydrogen. The remaining two electrons form a lone pair, which, as discussed earlier, significantly influences the molecule's geometry. The hybridization of orbitals in PH3 is a point of interest, as it deviates from the typical sp3 hybridization observed in similar tetrahedral molecules. Instead, PH3 exhibits a bond angle that suggests minimal or no hybridization, with the 3p orbitals of phosphorus participating in bond formation.
This bonding scenario in PH3 results in relatively weak P-H bonds compared to other hydrogen-containing compounds like ammonia (NH3). The bond strength is influenced by the larger atomic size of phosphorus and the decreased electronegativity compared to nitrogen. Consequently, PH3 is less stable than NH3 and more reactive, especially towards acids and oxidizing agents. Understanding the nature of bonding in PH3 is crucial for predicting its chemical behavior and potential reactivity in various chemical processes.
Lone Pairs and Their Effects
Lone pairs, or nonbonding electron pairs, play a pivotal role in shaping the electron pair geometry of molecules like PH3. These pairs occupy space around the central atom and exert repulsive forces on bonding pairs, thereby influencing the overall geometry and properties of the molecule. In PH3, the lone pair on phosphorus is responsible for the molecule's trigonal pyramidal shape, deviating from the ideal tetrahedral geometry.
The effects of lone pairs extend beyond geometry, impacting molecular polarity and reactivity. In PH3, the presence of a lone pair contributes to its polar nature, as the electron distribution is uneven. This polarity affects PH3's interactions with other molecules, influencing solubility, boiling point, and other physical properties. Moreover, the lone pair can participate in chemical reactions, acting as a nucleophile in certain circumstances.
Understanding the role of lone pairs is crucial in various fields, including chemistry, biochemistry, and materials science. By considering the influence of lone pairs, scientists can predict molecular behavior, design novel compounds, and tailor materials with specific properties. In the case of PH3, the lone pair's impact on geometry and reactivity provides valuable insights into the broader context of molecular interactions and chemical processes.
Hybridization in PH3
Hybridization is a concept that describes the mixing of atomic orbitals to form new hybrid orbitals, which are used in bonding. In many molecules, hybridization helps explain the observed shapes and bond angles. However, the case of PH3 presents an interesting deviation from typical hybridization patterns.
In PH3, the phosphorus atom uses its 3p orbitals to form bonds with hydrogen, with minimal or no mixing of the 3s orbital. This results in a bond angle of approximately 93.5°, which is smaller than the expected 109.5° seen in sp3 hybridized molecules. The reduced bond angle is due to the influence of the lone pair, which occupies more space and exerts greater repulsion on the bonding pairs.
The concept of hybridization in PH3 highlights the importance of considering both experimental data and theoretical models when analyzing molecular geometry. While hybridization provides a useful framework for understanding many molecules, exceptions like PH3 illustrate the complexity of chemical bonding and the need for a nuanced approach to molecular structure. By examining hybridization in PH3, we gain a deeper understanding of how atomic orbitals contribute to molecular shape and behavior.
Polarity of PH3
The polarity of a molecule is determined by its electron distribution and geometry. In the case of PH3, the presence of a lone pair on phosphorus results in an asymmetric electron distribution, giving the molecule a dipole moment and making it polar. This polarity is a key factor in defining the physical and chemical properties of PH3.
Polarity influences how PH3 interacts with other molecules, affecting its solubility, boiling point, and reactivity. As a polar molecule, PH3 can form dipole-dipole interactions with other polar substances, leading to moderate solubility in water and other polar solvents. However, its polarity is not as pronounced as in ammonia (NH3), where electronegativity differences between nitrogen and hydrogen enhance the dipole moment.
The polarity of PH3 also has implications for its chemical reactivity. The polar nature of PH3 enables it to participate in nucleophilic and electrophilic reactions, where the lone pair on phosphorus can act as a site for electron donation or acceptance. Understanding the polarity of PH3 is essential for predicting its behavior in chemical reactions and designing applications that exploit its unique properties.
Comparison with Other Molecules
Comparing PH3 with other molecules provides valuable insights into the influence of lone pairs and molecular geometry on chemical properties. One common point of comparison is with ammonia (NH3), a similar molecule in terms of composition but differing in behavior due to its geometry and electronegativity.
Both PH3 and NH3 have a similar trigonal pyramidal geometry due to the presence of a lone pair. However, the bond angles differ, with NH3 having a larger angle of about 107.5° compared to PH3's 93.5°. This difference is attributed to the smaller size and higher electronegativity of nitrogen, which leads to stronger N-H bonds and less distortion from the lone pair.
The differences in electronegativity also affect the polarity and chemical reactivity of these molecules. NH3 is more polar than PH3, resulting in higher solubility in water and stronger hydrogen bonding. In contrast, PH3 is less reactive and more stable, reflecting the weaker P-H bonds and larger atomic size of phosphorus.
By comparing PH3 with other molecules, we gain a deeper understanding of the factors that influence molecular geometry and properties. Such comparisons are essential for predicting the behavior of new compounds and designing materials with specific characteristics.
Applications of PH3
Phosphine (PH3) has a range of applications across various industries due to its unique chemical properties. One of its primary uses is in the semiconductor industry, where it serves as a dopant to modify the electrical properties of materials. Its ability to introduce phosphorus atoms into silicon and other substrates makes it a valuable tool in the production of electronic components.
In agriculture, phosphine is employed as a pesticide and fumigant to control pests in stored grain and other agricultural products. Its effectiveness in penetrating materials and killing insects without leaving harmful residues makes it a preferred choice for pest control. However, its toxicity requires careful handling and adherence to safety protocols to prevent exposure and environmental contamination.
Phosphine is also used in chemical synthesis as a reagent in various reactions. Its reactivity with acids and oxidizing agents allows it to participate in the formation of phosphorus-containing compounds, contributing to the development of pharmaceuticals, plastics, and other chemical products. Understanding the properties and behavior of PH3 is essential for optimizing its use in these applications and ensuring safe and efficient processes.
Frequently Asked Questions
What is the electron pair geometry of PH3?
The electron pair geometry of PH3 is trigonal pyramidal due to the presence of a lone pair on the phosphorus atom, which influences the arrangement of the bonding pairs.
How does the lone pair affect the geometry of PH3?
The lone pair on phosphorus in PH3 causes increased repulsion, pushing the hydrogen atoms closer together and resulting in a trigonal pyramidal shape with bond angles slightly less than 109.5°.
Is PH3 a polar molecule?
Yes, PH3 is a polar molecule. The asymmetric distribution of electrons due to the lone pair creates a dipole moment, contributing to its polar nature.
What is the hybridization of PH3?
PH3 exhibits minimal or no hybridization, with the phosphorus 3p orbitals participating in bonding, resulting in bond angles of approximately 93.5°.
How does PH3 compare to NH3 in terms of reactivity?
PH3 is less reactive than NH3 due to weaker P-H bonds and lower electronegativity of phosphorus. However, PH3 is more stable and less soluble in water compared to ammonia.
What are the applications of PH3?
PH3 is used in the semiconductor industry as a dopant, in agriculture as a pesticide and fumigant, and in chemical synthesis as a reagent for producing phosphorus-containing compounds.
Conclusion
Understanding the electron pair geometry of PH3 offers a window into the fascinating world of molecular chemistry. The unique trigonal pyramidal shape of PH3, influenced by its lone pair of electrons, provides insights into how molecular geometry affects physical properties and chemical reactivity. By examining the bonding, hybridization, and polarity of PH3, we gain a deeper appreciation for the complexities of chemical interactions and the principles governing molecular behavior.
As we explored throughout this article, PH3 serves as a valuable model for studying the effects of lone pairs and electron distribution on molecular geometry. Its applications in various industries, from semiconductors to agriculture, underscore the importance of understanding its properties to optimize its use and ensure safety. The knowledge gained from studying PH3 not only enriches our understanding of chemistry but also informs the development of new materials and technologies.
In conclusion, PH3 electron pair geometry is a testament to the intricate balance of forces that shape molecules and dictate their behavior. By continuing to explore and understand these principles, we can unlock new possibilities in science and technology, paving the way for innovations that harness the potential of chemical compounds like PH3.
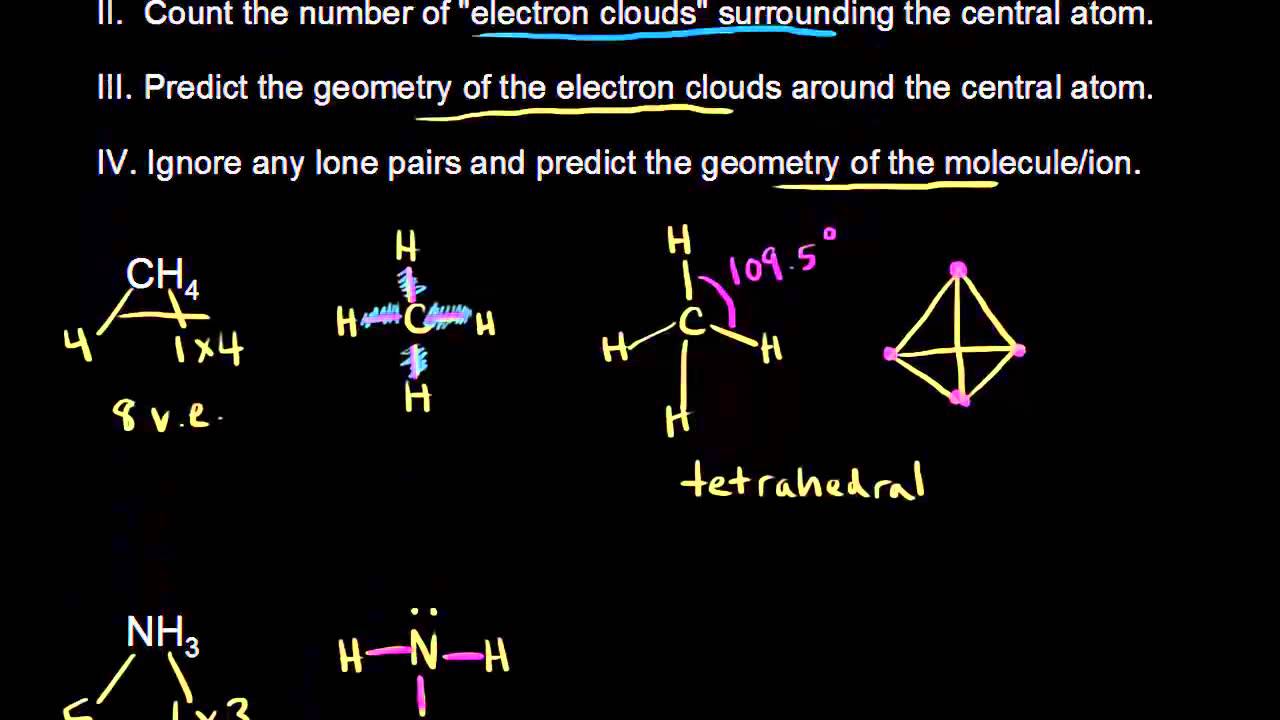
